Lipoproteins
Contents
Structure and Classification of Lipoproteins
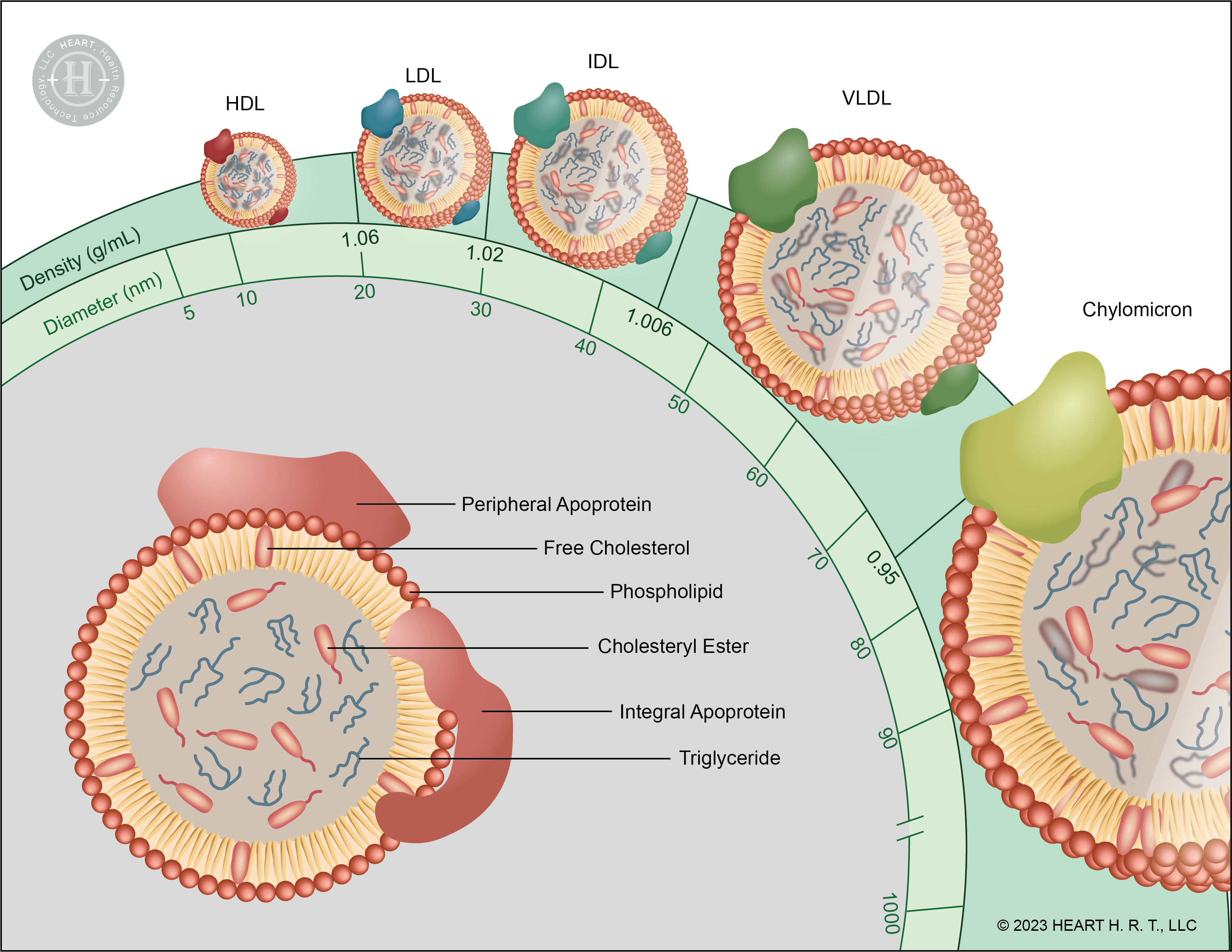
▲ Figure 1 : Structure and Classification of Lipoproteins
The structure of lipoproteins can be divided into the hydrophilic membrane and the central hydrophobic core. The hydrophilic membrane is composed of phospholipids, free cholesterol and apolipoproteins (Apo), while the central core contains cholesterol and triglycerides (TG). Lipoproteins are classified by density into chylomicrons (CM), very low-density lipoproteins (VLDL), intermediate-density lipoproteins (IDL), low-density lipoproteins (LDL) and high-density lipoproteins (HDL).
Low-Density Lipoprotein (LDL)
Low-density lipoproteins (LDL) are responsible for transporting cholesterol from the liver to body cells and tissues. When LDL binds to cholesterol, it forms "low-density lipoprotein-cholesterol (LDL-C)," also known as "low-density cholesterol." Numerous studies have shown that elevated levels of LDL-C are associated with increased risk of cardiovascular disease, leading LDL-C to be commonly referred to as "bad cholesterol" and serving as a widely used indicator of cardiovascular disease risk[1]. However, in some clinical cases, individuals may develop cardiovascular-related diseases such as atherosclerosis and angina pectoris despite having normal LDL-C levels in their medical reports[1][2]. Hence, LDL-C may not be the most accurate standard for predicting cardiovascular disease risk.
Mechanism of Hypolipidemic agents
Currently, there are various types of hypolipidemic agents in medical treatment, with the most common being "Statins" and "PCSK9 inhibitors." Statins inhibit the synthesis of cholesterol in the liver, while PCSK9 inhibitors enhance the efficiency of LDL-C degradation by binding with PCSK9.
Under normal circumstances, LDL-C binds to receptors on the surface of liver cells, known as Low-density lipoprotein receptors (LDLR), and is then transported into liver cells for degradation through endocytosis. Therefore, the quantity of LDLR is directly related to the concentration of LDL-C in the blood: the more LDLR present, the lower the concentration of LDL-C. PCSK9 (Proprotein Convertase Subtilisin/Kexin type 9), on the other hand, is a protein primarily synthesized in the liver. It selectively binds to LDLR and facilitates the degradation of both LDL-C and LDLR together. In summary, effectively inhibiting PCSK9 can prevent the degradation of LDLR, increasing their recycling rate, thereby improving the efficiency of LDL-C degradation[3].
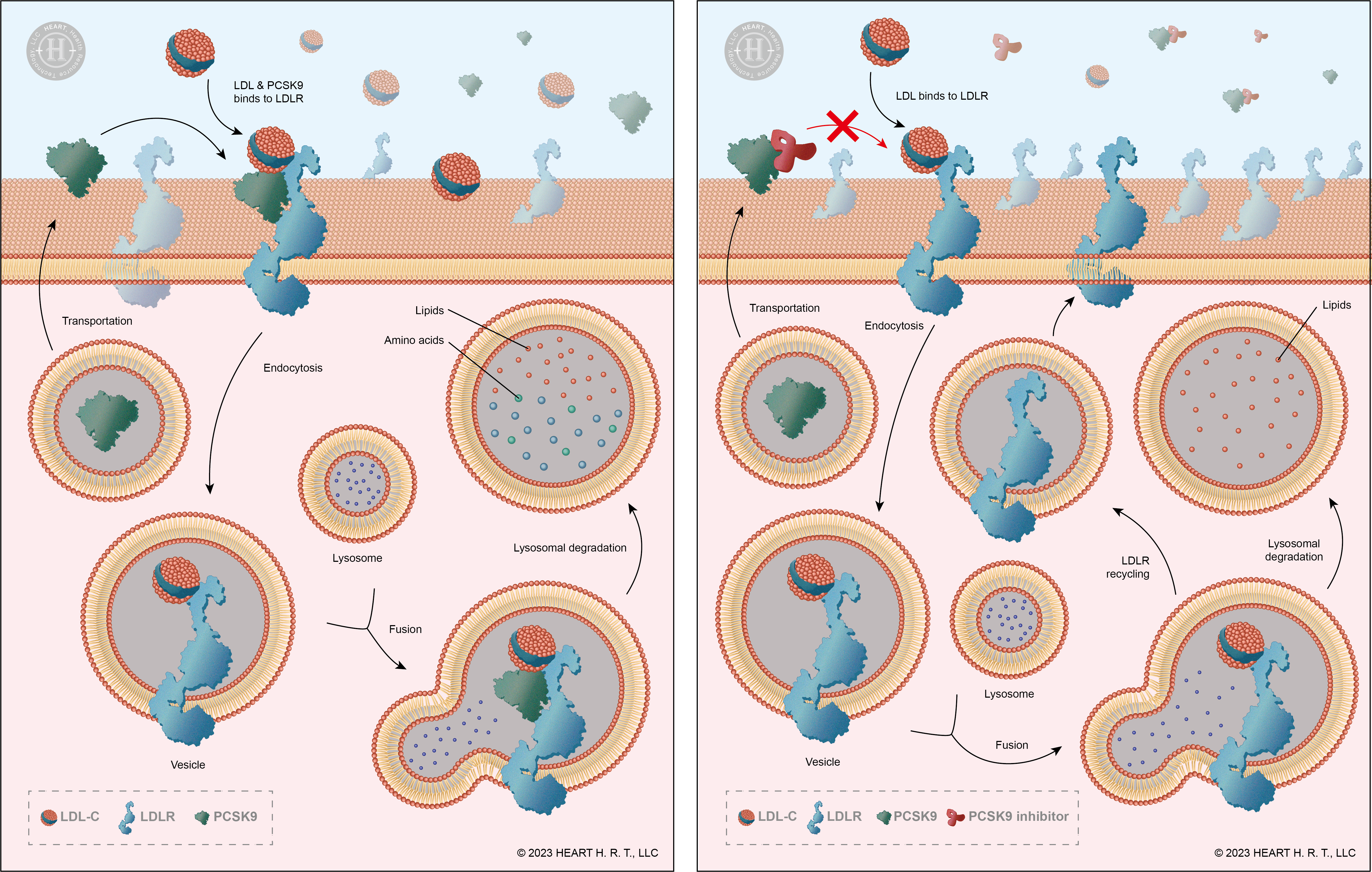
▲ Figure 2 : Mechanism of PCSK9 & PCSK9 Inhibitors
Alirocumab and Evolocumab are PCSK9 inhibitors. Clinical trial results indicate that although they can reduce overall LDL-C levels and decrease the risk of cardiovascular disease, their effectiveness is not as expected, and they have not caused a significant difference in the mortality rate of cardiovascular disease[4][5]. This suggests that in the LDL-C population, there may be some types that do not bind to LDLR and are not degraded.
The Most Electronegative Low-Density Lipoprotein: L5
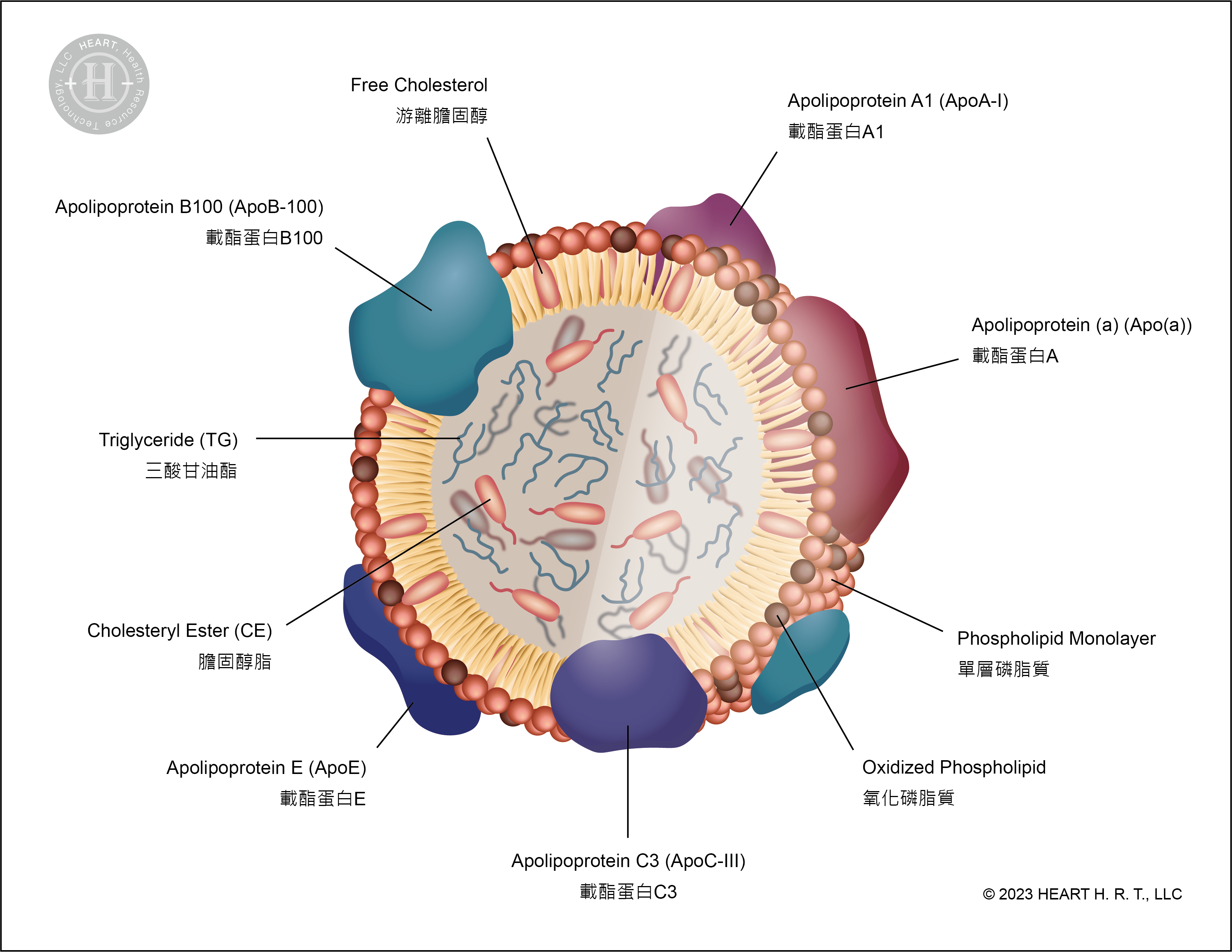
▲ Figure 3 : Structure of L5
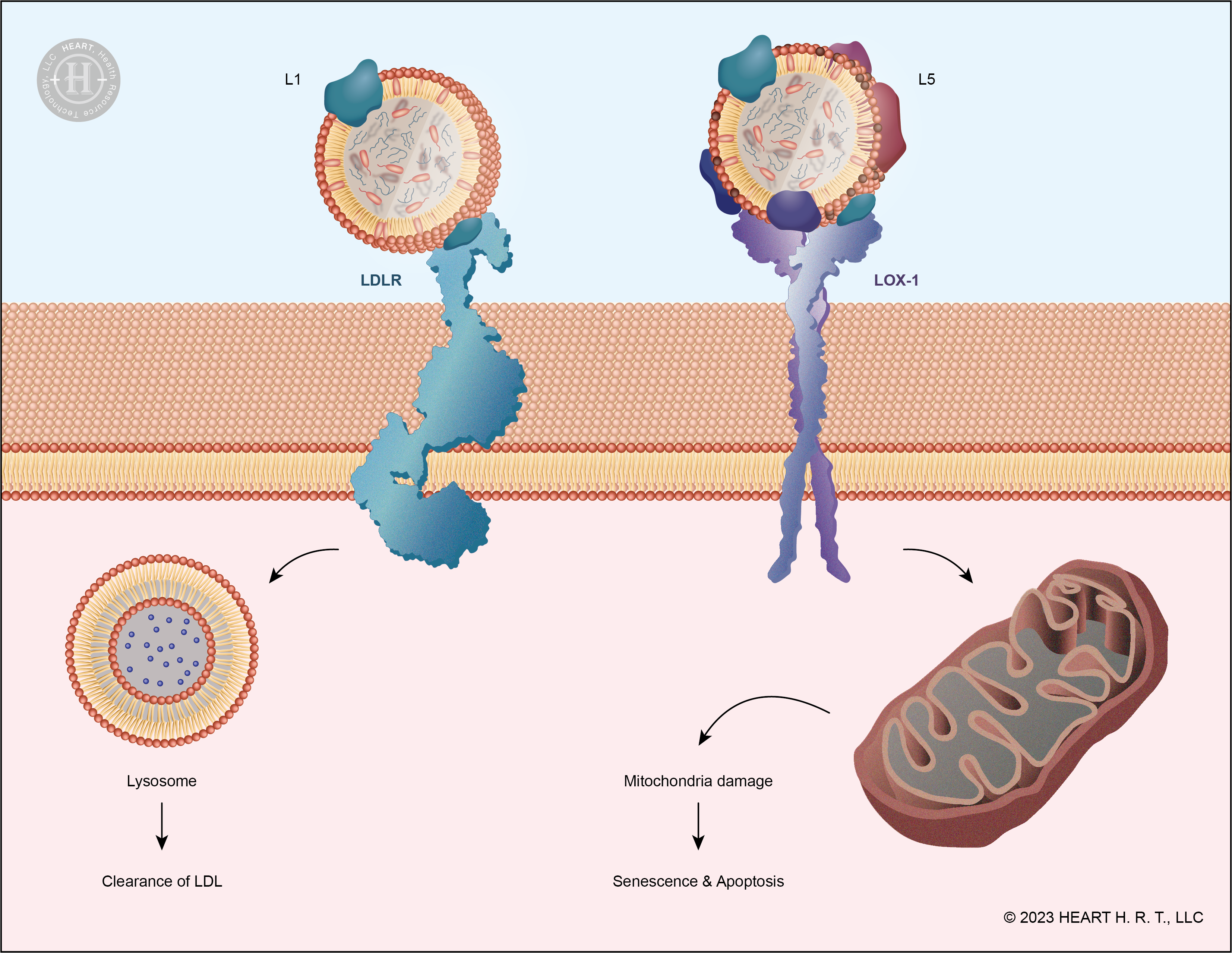
▲ Figure 4 : Receptors for L1 and L5: LDLR and LOX-1
The concept of electronegative low-density lipoprotein was first proposed by Professor Gotto from Baylor College of Medicine in 1979[6]. Its existence was further confirmed by another journal published in 1988[7]. In 2003, Professor Chu-Huang Chen (the founder of our company) published a paper in the journal Circulation , in which he classified LDL into five subfractions based on their levels of electronegativity: L1, L2, L3, L4, and L5. L1 is normal LDL, while L5 has the highest electronegativity and is the most prone to oxidation, making it a major contributor to atherosclerosis and other cardiovascular diseases[2][8][9].
Structurally, Apolipoprotein B100 (ApoB-100) constitutes approximately 99.71% of Apo in normal LDL (L1). In contrast, ApoB-100 only accounts for 61.26% of Apo in L5, with the remaining portion comprising Apolipoprotein a (Apo(a)), Apolipoprotein A1 (ApoA1), Apolipoprotein C3 (ApoCIII) and Apolipoprotein E (ApoE). This composition results in L5 carrying more negative charges[9].
In the process of L1 binding with LDLR, the positively charged ε-amino group (NH3+) on ApoB-100 plays a crucial role. L1 interacts with negatively charged residues on LDLR through this amino group and is then internalized into the cell via endocytosis and degraded. However, L5 carries more negative charges, resulting in a low affinity for LDLR due to its conformation, and thus it is not recognized by LDLR[9]. Unlike L1, L5 binds to the LOX-1 (Lectin-like oxidized LDL receptor) on the cell surface, initiating downstream signaling pathways that lead to cell apoptosis. The accumulation of dead cells in the vascular walls narrows the vessel diameter, contributing to the development of cardiovascular diseases.
High-Density Lipoprotein (HDL)
High-density lipoprotein (HDL) is a type of lipoprotein responsible for transporting cholesterol back to the liver from the bloodstream. When bound to cholesterol, HDL forms "high-density lipoprotein cholesterol (HDL-C)," also known as "good cholesterol." Numerous epidemiological studies have shown a significant negative correlation between HDL-C and cardiovascular diseases. Therefore, HDL-C is often referred to as "good cholesterol" and has become a common component of modern lipid profile tests, serving as one of the indicators for cardiovascular disease assessment. However, in recent years, an increasing number of research findings have suggested that HDL-C is not a reliable indicator for preventing cardiovascular diseases[10][11][12][13][14].
Cholesteryl Ester Transfer Protein (CETP)
Cholesteryl ester transfer protein (CETP) is a protein involved in the cholesterol metabolism pathway. Its function is to transfer cholesteryl esters (CE) from HDL to very low-density lipoprotein (VLDL) and LDL, while transferring triglycerides carried by VLDL and LDL to HDL[10][12]. Therefore, effective inhibition of CETP can lead to an increase in the concentration of "good cholesterol" in the blood.
Scientists used three medications, Torcetrapib[10], Dalcetrapib[11] and Evacetrapib[13] , to inhibit CETP. They administered these medications to patients with acute coronary syndrome and individuals at high risk of cardiovascular diseases. The experimental results showed that although the concentration of HDL increased significantly, there was no reduction in the cardiovascular disease risk of the patients due to the increase in HDL concentration[10][11][13]. In an experiment by Furtado and Sacks et al. published in ATVB (2022), it was found that the CETP inhibitors Torcetrapib and Evacetrapib increased the concentration of apolipoprotein A1 (ApoA-I), especially in HDL subfractions containing apolipoprotein C-III (ApoC-III) in their structure, thus increasing the risk of cardiovascular diseases[14].
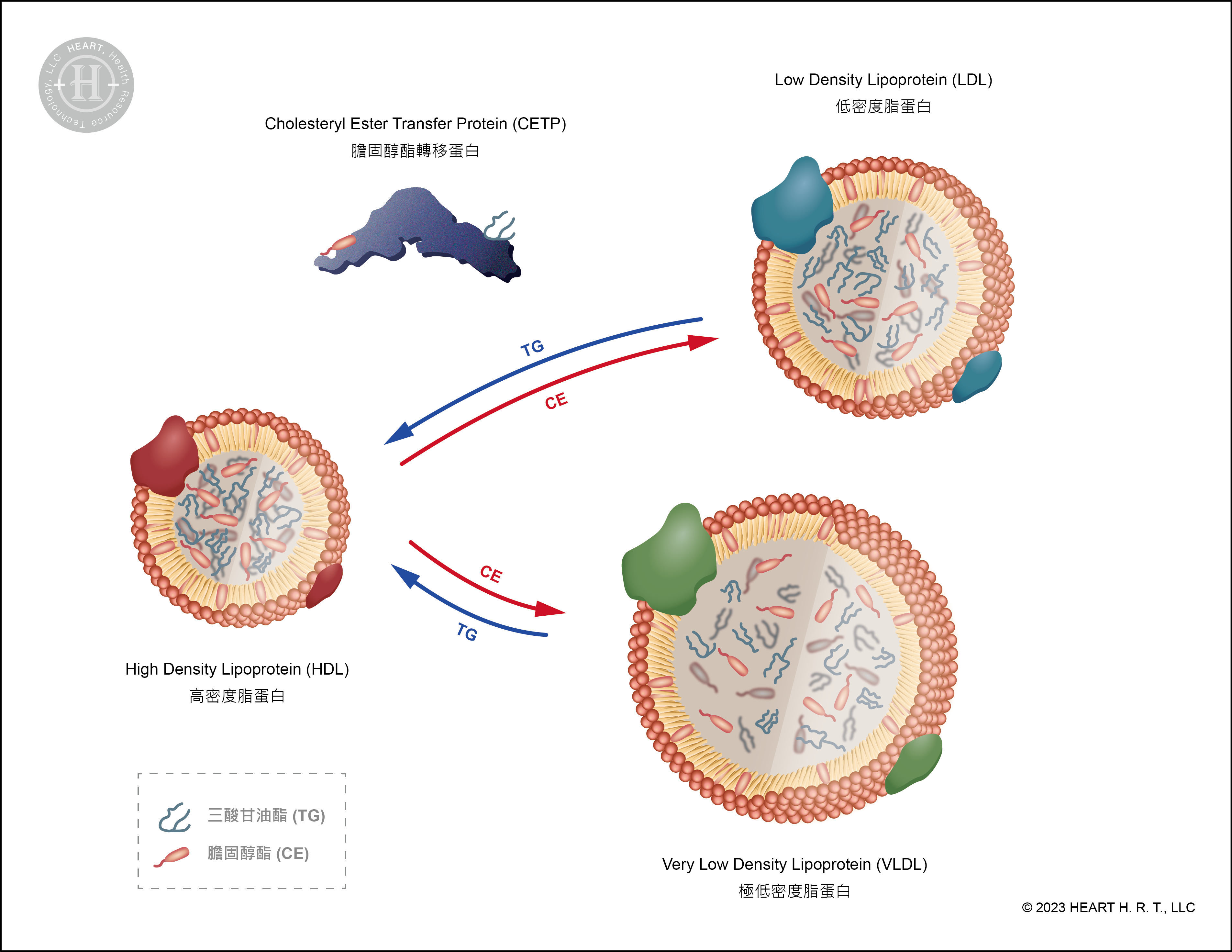
▲ Figure 5 : Mechanism of CETP
The Most Electronegative High-Density Lipoprotein: H5
HDL can be divided into five subfractions based on the magnitude of their charge, namely H1 through H5, with H5 being the subfraction with the highest negative charge. ApoC-III, furthermore, is primarily expressed on top of H5[14][15]. Additionally, related research indicates that ApoC-III is one of the indicators of HDL dysfunction[12]. Therefore, accurately measuring the concentration of H5 in plasma would be a more effective standard for assessing cardiovascular disease risk compared to measuring HDL-C.
Citation
[1] Ke, L. Y., Stancel, N., Bair, H., Chen, C. H. 2014. The Underlying Chemistry of Electronegative LDL’s Atherogenicity. Curr Atheroscler Rep. 16:428.
[2] Wang, G. J., Chang, C. T., Yang, C. H., Chen, C. H. 2012. Negatively charged L5 as a naturally occurring atherogenic low-density lipoprotein. BioMedicine. 2(4):147-154.
[3] Shimada, Y. J., Cannon, C. P. PCSK9 (Proprotein convertase subtilisin/kexin type 9) inhibitors: past, present, and the future. European Heart Journal, 36(36):2415–2424.
[4] Karatasakis, A., Danek, B. A., Karacsonyi, J., Rangan, B. V., Roesle, M. K., Knickelbine, T., Miedema, M. D., Khalili, H., Ahmad, Z., Abdullah, S., Banerjee, S., Brilakis, E. S. 2017. Effect of PCSK9 Inhibitors on Clinical Outcomes in Patients With Hypercholesterolemia: A Meta‐Analysis of 35 Randomized Controlled Trials. J Am Heart Assoc. 9;6(12):e006910.
[5] Navarese, E. P., Robinson, J. G., Kowalewski, M., Kolodziejczak, M., Andreotti, F., Bliden, K., Tantry, U., Kubica, J., Raggi, P., Gurbel, P. A. 2018. Association Between Baseline LDL-C Level and Total and Cardiovascular Mortality After LDL-C Lowering: A Systematic Review and Meta-analysis. JAMA. 319(15):1566-1579.
[6] Hoff, H. F., Bradley, W. A., Heideman, C. L., Gaubatz, J. W., Karagas, M. D., Gotto Jr, A. M. 1979. Characterization of low density lipoprotein-like particle in the human aorta from grossly normal and atherosclerotic regions. Biochim Biophys Acta. 573(2):0–374.
[7] Avogaro, P., Bon, G. B., Cazzolato, G. 1988. Presence of a modified low density lipoprotein in humans. Arteriosclerosis. 8(1):79-87.
[8] Chen, C. H., Jiang, T., Yang, J. H., Jiang, W., Lu, J., Marathe, G. K., Pownall, H. J., Ballantyne, C. M., McIntyre, T. M., Henry, P. D., Yang, C. H. 2003. Low-Density Lipoprotein in Hypercholesterolemic Human Plasma Induces Vascular Endothelial Cell Apoptosis by Inhibiting Fibroblast Growth Factor 2 Transcription. Circulation. 107:2102–2108.
[9] Ke, L. Y., Engler, D. A., Lu, J., Matsunami, R. K., Chan, H. C., Wang, G. H., Yang, C. H., Chang, J. H., Chen, C.H. 2011. Chemical composition–oriented receptor selectivity of L5, a naturally occurring atherogenic low-density lipoprotein. Pure Appl Chem. 83(9):10.
[10] Barter, P. J., Caulfield, M., Eriksson, M., Grundy, S. M., Kastelein, J. J., Komajda, M., Lopez-Sendon, J., Mosca, L., Tardif, J. C., Waters, D. D., Shear, C. L., Revkin, J. H., Buhr, K. A., Fisher, M. R., Tall, A. R., Brewer, B. 2007. Effects of Torcetrapib in Patients at High Risk for Coronary Events. N Engl J Med. 22;357(21):2109-22.
[11] Schwartz, G. G., Olsson, A. G., Abt, M., Ballantyne, C. M., Barter, P. J., Brumm, J., Chaitman, B.R., Holme, I. M., Kallend, D., Leiter, L. A., Leitersdorf, E., McMurray, J. J. V., Mundl, H., Nicholls, S. J., Shah, P. K., Tardif, J. C., Wright, R. S. 2012. Effects of Dalcetrapib in Patients with a Recent Acute Coronary Syndrome. N Engl J Med. 367:2089-2099.
[12] Zheng, C., Aikawa, M. 2012. High-Density Lipoproteins: From Function to Therapy. Journal of the American College of Cardiology (JACC). 60(23): 2380-2383.
[13] Lincoff, A. M., Nicholls, S. J., Riesmeyer, J. S., Barter, P. J., Brewer, H. B., Fox, K. A. A., Gibson, C. M., Granger, C., Menon, V., Montalescot, G., Rader, D., Tall, A. R., McErlean, E., Wolski, K., Ruotolo, G., Vangerow, B., Weerakkody, G., Goodman, S. G., Conde, D., McGuire, D. K., Nicolau, J. C., Leiva-Pons, J. L., Pesant, Y., Li, W., Kandath, D., Kouz, S., Tahirkheli, N., Mason, D., Nissen, S. E. 2017. Evacetrapib and Cardiovascular Outcomes in High-Risk Vascular Disease. N Engl J Med. 18;376(20):1933-1942.
[14] Furtado, J. D., Ruotolo, G., Nicholls, S. J., Dullea, R., Carvajal-Gonzalez, S., Sacks, F. M. 2022. Pharmacological Inhibition of CETP (Cholesteryl Ester Transfer Protein) Increases HDL (HighDensity Lipoprotein) That Contains ApoC3 and Other HDL Subspecies Associated with Higher Risk of Coronary Heart Disease. Arterioscler Thromb Vasc Biol. 42:227–237.
[15] Hsieh, J. Y., Chang, C. T., Huang, M. T., Chang, C. M., Chen, C. Y., Shen, M. Y., Liao, H. Y., Wang, G. J., Chen, C. H., Chen, C. J., Yang, C. Y. 2013. Biochemical and Functional Characterization of Charge-Defined Subfractions of High-Density Lipoprotein from Normal Adults. Analytical Chemistry. 85(23):11440–11448.